Polymerase chain reaction (PCR) is widely used across life science research laboratories as a technique to rapidly and efficiently amplify a small amount of DNA to make millions of copies (Fig. 1). PCR was first invented in the 1980s by Kary Mullis, who won the Nobel Prize in Chemistry in 1993 for his pioneering work inventing this technique1,2.
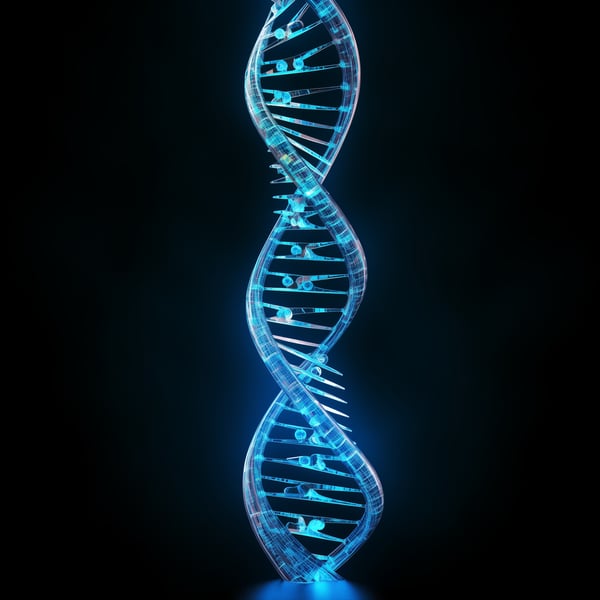
Figure 1. PCR supports the rapid amplification of one or a few copies of a DNA sequence into millions of copies.
PCR's high specificity, sensitivity, and efficiency have quickly established it as one of the most widely used laboratory techniques, with applications spanning a broad range of fields. Scientists use PCR in research labs to study genes and their functions, including cloning, gene expression, genotyping, sequencing, and mutagenesis3. PCR is also a vital diagnostic tool for detecting genetic diseases and pathogens and is crucial in forensic science for DNA amplification4,5.
Despite its remarkable capabilities, PCR does have its kryptonite. Manual PCR workflows can be slow and error-prone, hindering research progress and extending turnaround times. PCR workflow optimization is crucial to overcome these challenges. Here, we explore the pivotal role of liquid handling in PCR workflow optimization to help you maximize the efficiency and reliability of your PCR processes.
Mastering Liquid Handling Techniques
PCR workflows involve numerous liquid handling steps, often requiring the transfer of tiny liquid volumes. These steps can present challenges related to efficiency, accuracy, and contamination. However, PCR workflow optimization, specifically mastering liquid handling techniques and selecting appropriate tools, can mitigate many of these issues6. To minimize these challenges, it is essential that researchers focus on the following components:
- Accurate pipetting techniques. Mastering precise pipetting techniques is crucial for accurately transferring small liquid volumes, which helps ensure the correct reagent concentrations and successful PCR amplification.
- Minimizing errors. Using high-quality pipettes and regularly deep cleaning and calibrating them can significantly reduce the risk of errors, such as incorrect volume measurements or cross-contamination, thereby enhancing data integrity.
- Maintaining consistency. Consistent pipetting practices, such as using the same pipetting speed and angle, help ensure uniformity across samples, which is essential for reproducible and comparable PCR data across different experiments.
Limitations of Manual Liquid Handling
Unfortunately, even the most skilled liquid handler often faces challenges with PCR workflow optimization, which can be attributed to the inherent limitations of manual liquid handling. Manual pipetting is highly time-consuming and introduces inefficiencies, even for the most efficient scientists. It is also a monotonous task that requires minimal research or analytical skills, wasting valuable research time. These issues are exacerbated when handling large numbers of samples, as scalability limitations can hinder project progress. Moreover, the repetitive nature of pipetting in PCR workflows, especially with numerous samples, can lead to repetitive strain injury (RSI).
How Automation Helps
To overcome these significant challenges, introducing an automated pipetting setup like the I.DOT Liquid Handler into PCR workflows is highly effective (Fig. 2).
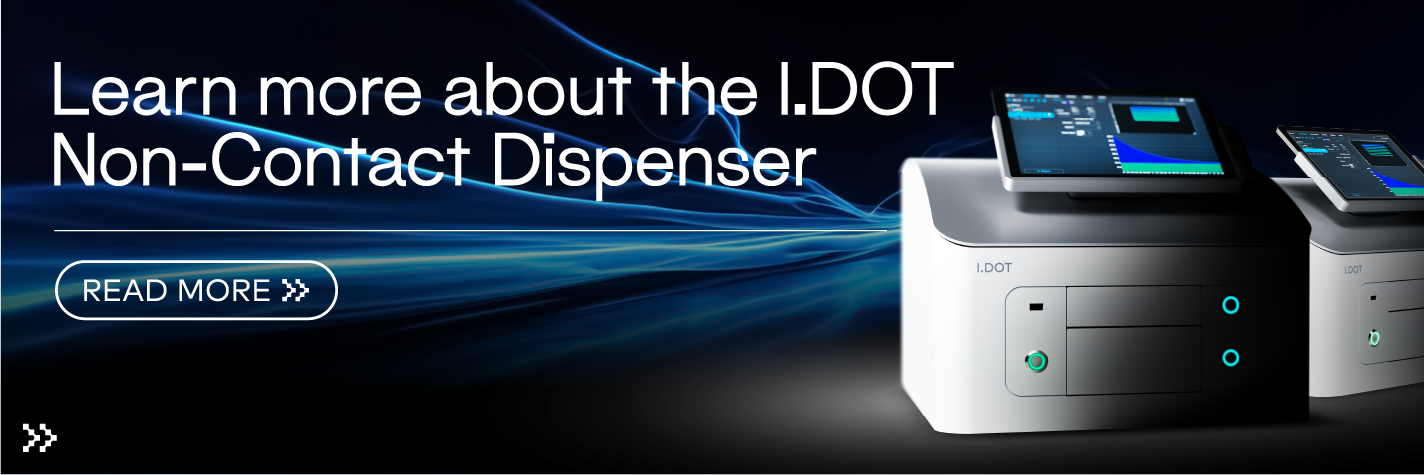

Figure 2. The I.DOT Non-Contact Dispenser supports the automation of PCR liquid handling steps for PCR workflow optimization.
Automation offers numerous key benefits for PCR workflow optimization, including:
- Improved accuracy and reproducibility. Automated pipetting systems, such as the I.DOT Non-Contact Dispenser, ensure precise liquid handling, significantly reducing human error and enhancing the reproducibility of PCR results, which is crucial for reliable data.
- Increased efficiency and throughput. Automation streamlines PCR workflows by handling multiple samples simultaneously and performing pipetting tasks faster than manual processes, thereby increasing throughput and enabling high-volume processing.
- Reduced risk of RSI. By automating repetitive pipetting tasks, scientists are spared from tedious manual pipetting steps, reducing RSI and promoting a safer working environment.
- Scalability and flexibility. Automated systems can easily be scaled to accommodate varying numbers of samples and adapted to different PCR protocols, supporting a wide range of experimental designs.
Conclusion
Mastering liquid handling techniques and integrating automation can improve your PCR workflow optimization, significantly enhancing accuracy, efficiency, and consistency while reducing errors and physical strain. The use of automated pipetting systems, such as the I.DOT Non-Contact Dispenser, addresses the limitations of manual pipetting, offering improved reproducibility and scalability and reducing the burden on research staff and students. By embracing these strategies, laboratories can maximize the potential of PCR, ensuring robust and reliable results across various applications.
Struggling with manual liquid handling in your PCR experiments?
There might be a better way! Book a demo and discover how automation can revolutionize your workflows and empower you to become a PCR master.
References
- Saiki RK, Scharf S, Faloona F, et al. Enzymatic Amplification of β-Globin Genomic Sequences and Restriction Site Analysis for Diagnosis of Sickle Cell Anemia. Science. 1985;230(4732):1350-1354. doi:10.1126/science.2999980
- The Nobel Prize in Chemistry 1993. NobelPrize.org. Accessed June 1, 2023. https://www.nobelprize.org/prizes/chemistry/1993/mullis/facts/
- Hoseini S, Sauer MG. Molecular cloning using polymerase chain reaction, an educational guide for cellular engineering. J Biol Eng. 2015;9(1):2. doi:10.1186/1754-1611-9-2
- Yang S, Rothman RE. PCR-based diagnostics for infectious diseases: uses, limitations, and future applications in acute-care settings. Lancet Infect Dis. 2004;4(6):337-348. doi:10.1016/S1473-3099(04)01044-8
- Morling N. PCR in forensic genetics. Biochem Soc Trans. 2009;37(2):438-440. doi:10.1042/BST0370438
- Lorenz TC. Polymerase Chain Reaction: Basic Protocol Plus Troubleshooting and Optimization Strategies. J Vis Exp. 2012;(63):3998. doi:10.3791/3998