Quantitative polymerase chain reaction (qPCR) is a powerful technique that allows scientists to quantify DNA in a sample. By tagging DNA molecules with a fluorescent dye, qPCR enables real-time tracking and measurement of PCR product formation. The fluorescence signal increases proportionally with the amount of DNA produced in each cycle, allowing for precise and highly sensitive quantification compared to a standard curve of DNA with known concentrations1,2.
qPCR has quickly become a cornerstone of modern research, owing to its high sensitivity, precision, and versatility. It plays a crucial role in enabling scientists to explore gene expression patterns, uncover genetic variations, and detect pathogens with a remarkably high degree of sensitivity. Additionally, it is important in cancer research, helping clinical research teams to identify biomarkers and monitor disease progression. It is also essential in drug development, where it enables researchers to assess the impact of new treatments on gene expression.
Notwithstanding its widespread applications, qPCR assays can be challenging to perform and come with numerous obstacles. Often, achieving successful results requires effective qPCR troubleshooting3. Here, we will explore common challenges and qPCR troubleshooting strategies to overcome them.
Common qPCR Issues & Troubleshooting Tips
Low Yield
One of the most common challenges in qPCR workflows is low yield, which indicates suboptimal reaction efficiency and often results in less quantitative data. This frustrating issue can arise from several factors, including poor RNA quality, inefficient cDNA synthesis, and suboptimal primer design.
Improving RNA quality involves optimizing RNA purification steps and performing appropriate clean-up procedures thoroughly to ensure high integrity and the absence of inhibitors4. Effective qPCR troubleshooting also requires adjusting cDNA synthesis conditions and ensuring consistent reagent volumes in the reaction. Finally, optimizing primers using specialized design software can help ensure they have the appropriate length, GC content, and melting temperature (Tm), while also checking for potential secondary structures or dimer formation5.
Non-Specific Amplification
Another frequent challenge experienced by even the most seasoned scientists is non-specific amplification. This occurs, more often than not, due to the formation of primer dimers or primer-template mismatches5.
To address this, researchers can redesign primers using specialized software to identify and avoid potential primer dimers. If primer redesign is not feasible, optimizing the annealing temperature can reduce the risk of non-specific amplification.
Ct Value Variations
Last but not least, a molecular biologist's nightmare: Ct value variations. Despite their prevalence, Ct value variations tend to be easily manageable. These variations, typically caused by manual errors such as inconsistent pipetting, can lead to differences in template concentrations across assays, requiring extensive qPCR troubleshooting procedures. Ensuring proper pipetting techniques can enhance consistency and reduce Ct value variability. Moreover, using reliable liquid handling systems or, better still, automated systems can further simplify this process and improve reproducibility.
Benefits of Automation in qPCR Workflows
1. Improved Accuracy & Reproducibility
Automated pipetting systems effectively enhance precision in liquid handling, significantly reducing human error and boosting the quantifiability and reproducibility of results without the need for extensive qPCR troubleshooting. This is especially beneficial when assays are regularly performed by different users.
2. Reduced Contamination Risk
Given qPCR's high sensitivity, preventing contamination is crucial. Using an automated dispensing system, particularly one with a closed system, minimizes the risk of cross-contamination, thereby addressing a major challenge in sample handling.
3. Increased Throughput & Efficiency
Automation increases efficiency in qPCR workflows by enabling parallel processing of multiple samples and performing pipetting tasks faster than manual methods, as evidenced in a recent study comparing manual versus automated approaches to gene expression quantification in a marine model6. This not only frees up scientists for other tasks but also allows for higher throughput and scalability of experiments (Fig. 1).

Figure 1. qPCR workflows often involve numerous pipetting steps that can be challenging to perform manually, particularly when scaling up to a higher throughput setup.
Introducing the I.DOT Liquid Handler
The I.DOT Liquid Handler is designed to tackle all your qPCR challenges with several standout features (Fig. 2). This high-precision liquid handling system is compatible with both 96- and 384-well qPCR plates. Its compact size allows for easy positioning in the lab, and it can be moved as needed to integrate seamlessly with different workflows, equipment, or users.
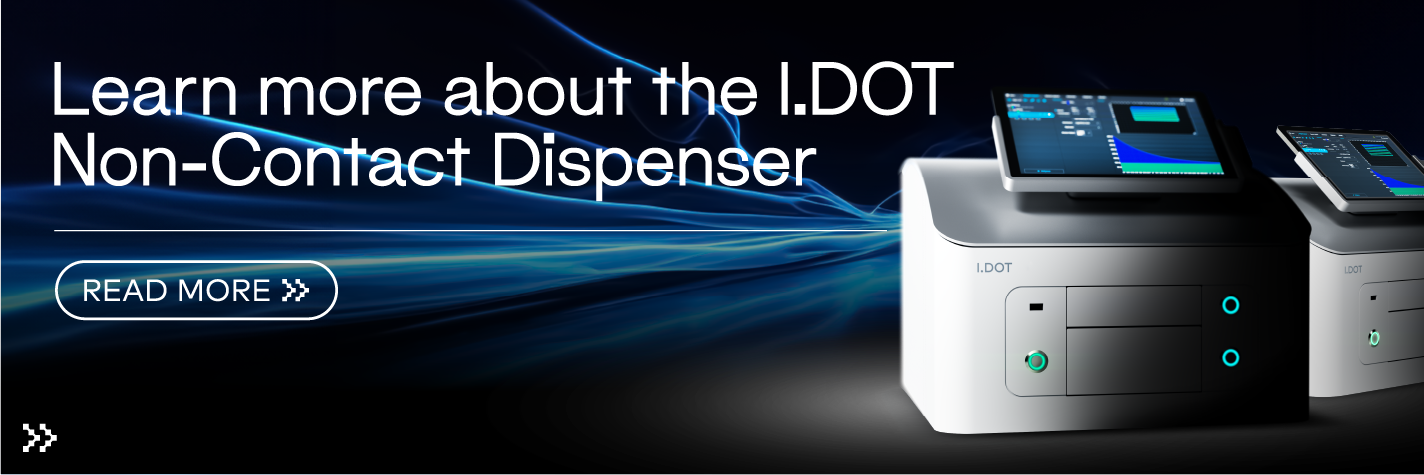
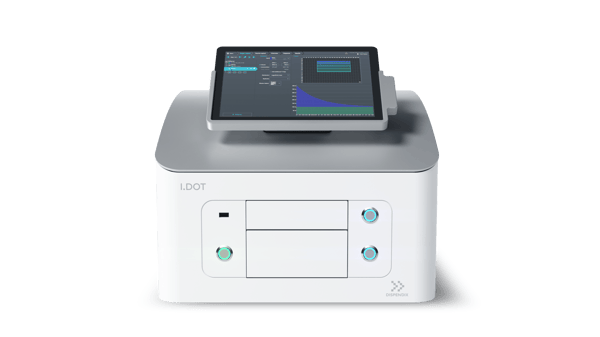
Figure 2. The I.DOT Non-Contact Dispenser is equipped with several features that overcome the common challenges researchers face with qPCR.
With a closed, tipless system, the I.DOT Non-Contact Dispenser minimizes the risk of cross-contamination. It has exceptional precision and is capable of accurately handling volumes as low as 4 nL, ensuring consistent Ct values across experimental replicates. Additionally, its built-in volume verification provides users with confidence in their assay success and result validity.
Conclusion
The sensitivity, precision, and versatility of qPCR render it an indispensable tool in gene expression analysis, pathogen detection, and drug development, to name but a few. Despite its advantages, qPCR presents several challenges, such as low yield, non-specific amplification, and Ct value variations. Implementing proper qPCR troubleshooting strategies and leveraging automated systems, like the I.DOT Non-Contact Dispenser, can significantly enhance qPCR workflows by improving accuracy, reducing contamination risks, and increasing efficiency.
Frustrated with inconsistent qPCR results?
The I.DOT Liquid Handler can help! Book a demo today and discover how automation with I.DOT can ensure successful experiments.
References
- Kralik P, Ricchi M. A Basic Guide to Real Time PCR in Microbial Diagnostics: Definitions, Parameters, and Everything. Front Microbiol. 2017;8. doi:10.3389/fmicb.2017.00108
- Schmittgen TD, Zakrajsek BA, Mills AG, Gorn V, Singer MJ, Reed MW. Quantitative Reverse Transcription–Polymerase Chain Reaction to Study mRNA Decay: Comparison of Endpoint and Real-Time Methods. Anal Biochem. 2000;285(2):194-204. doi:10.1006/abio.2000.4753
- Lorenz TC. Polymerase Chain Reaction: Basic Protocol Plus Troubleshooting and Optimization Strategies. J Vis Exp. 2012;(63):3998. doi:10.3791/3998
- Schrader C, Schielke A, Ellerbroek L, Johne R. PCR inhibitors - occurrence, properties and removal. J Appl Microbiol. 2012;113(5):1014-1026. doi:10.1111/j.1365-2672.2012.05384.x
- Delghandi M, Delghandi MP, Goddard S. The Significance of PCR Primer Design in Genetic Diversity Studies: Exemplified by Recent Research into the Genetic Structure of Marine Species. In: Basu C, ed. PCR Primer Design. Vol 2392. Methods in Molecular Biology. Springer US; 2022:3-15. doi:10.1007/978-1-0716-1799-1_1
- Annona G, Liberti A, Pollastro C, Spagnuolo A, Sordino P, De Luca P. Reaping the benefits of liquid handlers for high-throughput gene expression profiling in a marine model invertebrate. BMC Biotechnol. 2024;24(1):4. doi:10.1186/s12896-024-00831-y